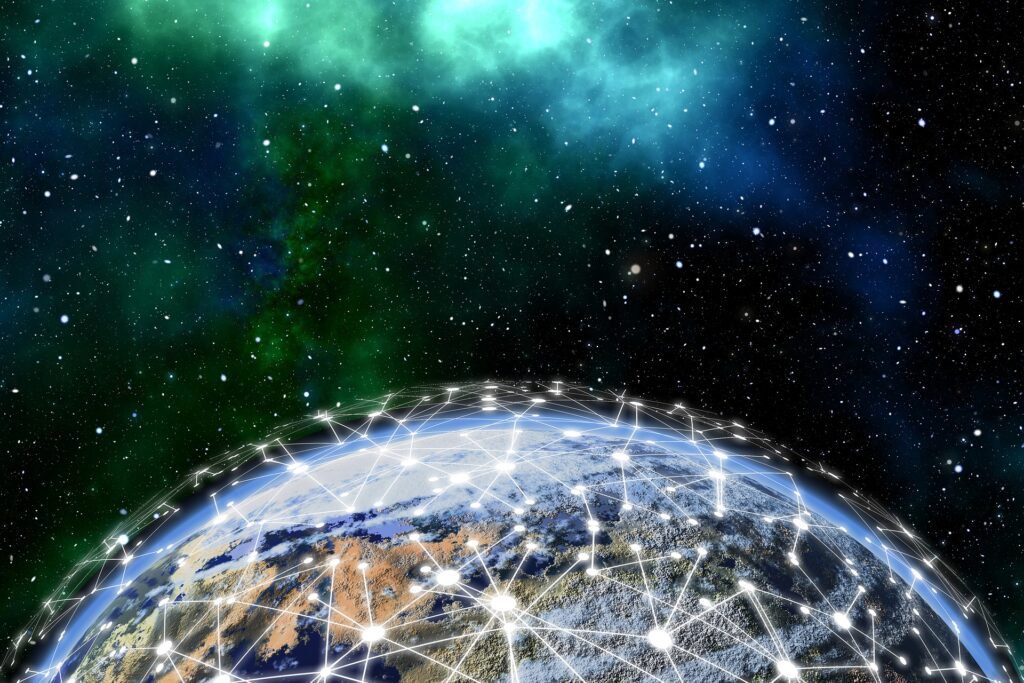
Members Involved
- Narayanan Rengaswamy
- Nithin Raveendran
- Bane Vasic
- Filip Rozpędek
- Liang Jiang
- Michele Pacenti
Project Summary
The quantum internet will be a network of quantum systems hosted at end nodes, interconnected through quantum repeaters. The quantum operations performed at these nodes and repeaters, as well as the physical channels connecting them, will be noisy. The physical carriers of quantum information, namely qubits, will also be fragile and susceptible to this noise. Hence, we need to continually detect and correct errors in all parts of the network to guarantee its robustness. In this project, we develop quantum error correction (QEC) based techniques to perform critical network tasks such as distributing entanglement between the nodes. We also integrate QEC methods with the functions of the quantum repeaters to ensure resilience to noise.
Project Outline
The University of Arizona leads the NSF-funded Engineering Research Center called the Center for Quantum Networks (CQN), whose goal is to lay the foundations of the quantum internet. Entanglement is a key feature of the quantum regime that distinguishes the quantum internet from the classical internet. Hence, distributing high-quality entangled states between nodes of the network becomes a quintessential task of the network. Since the communication channels connecting the nodes are noisy, we have developed a protocol that uses quantum error correction (QEC) to distill high quality entanglement from the initially distributed noisy entangled states. Currently, the protocol assumes that all quantum operations performed at network nodes are perfect, and only the communication channels between the nodes introduce noise. However, part of the goals for this project is to assume realistic quantum operations and noise models, and adapt the protocol to such settings.
In a quantum network, the end nodes are likely far apart. Since quantum information quickly decays when transmitted over large distances, we need to build so-called quantum repeaters and place them in the network for receiving and forwarding quantum signals. The quantum operations that these repeaters perform are themselves unreliable and noisy. Hence, QEC is essential for robust functioning of these repeaters. We have developed a QEC technique that concatenates physical-level continuous variable qubit encodings, called the Gottesman-Kitaev-Preskill (GKP) code, with an outer discrete variable code, and shown that such a technique is very promising to quickly and efficiently correct errors. In this project, we will explore such concatenation schemes under more realistic noise, and investigate how to integrate them with other network protocols.
Project Media
Talks
N. Rengaswamy, A. Raina, N. Raveendran, B. Vasić, “Distilling GHZ states using stabilizer codes,” in Proc. Beyond IID in Information Theory Workshop, Sep 2021. [Online] Available: https://www.youtube.com/watch?v=Bl8BKnuntTA
N. Rengaswamy, A. Raina, N. Raveendran, and B. Vasić, “Distilling GHZ states using stabilizer codes,” Invited Talk at Duke QEC Seminar, Duke University, Sep. 16 2021
N. Raveendran, F. Rozpedek, N. Rengaswamy, A. Raina, L. Jiang, and B. Vasić, “Finite rate QLDPC-GKP coding scheme that surpasses the CSS Hamming bound,” Invited Talk at QCDA Seminar, Jan. 10 2022
Publications
N. Rengaswamy, A. Raina, N. Raveendran, B. Vasić, “Distilling GHZ states using stabilizer codes,” arXiv preprint arXiv:2109.06248, 2021. Implementation: https://github.com/nrenga/ghz_distillation_qec
N. Rengaswamy, A. Raina, N. Raveendran, B. Vasić, “Distillation of multi-qubit GHZ states using stabilizer codes,” submitted to IEEE International Symposium on Information Theory (ISIT), 2022
N. Raveendran, N. Rengaswamy, F. Rozpędek, A. Raina, L. Jiang, B. Vasić, “Finite rate QLDPC-GKP coding scheme that surpasses the CSS Hamming bound,” arXiv preprint arXiv:2111.07029, 2021

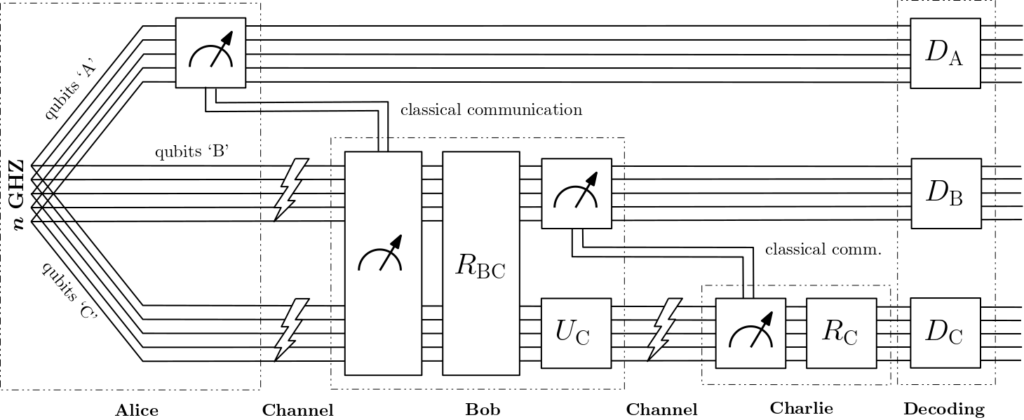